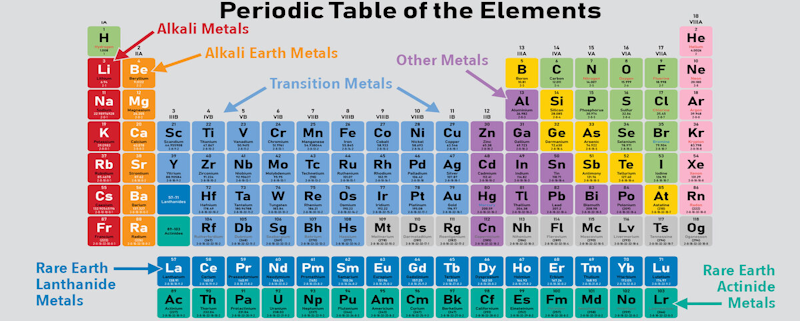
Gold, highly valued and widely used, displays a set of chemical properties that sets it apart from other elements, including metals. Here is a list of some of the chemical properties of gold:
- Atomic Number: 79
- Symbol: Au
- Atomic Mass: 196.967 amu
- Electron Configuration: [Xe] 4f14 5d10 6s1
- Oxidation States: +1 (aurous), +3 (auric)
- Density: 19.32 g/cm³
- Melting Point: 1064.18°C (1947.52°F)
- Boiling Point: 2856°C (5173°F)
With chemical symbol “Au”, deriving from the Latin word aurum, which means shining dawn. All of gold properties make it very useful in various areas: jewelry, electronics, medicine and more.
Gold Atomic Structure
Gold has the atomic number 79, placing it in Group 11 of the periodic table, alongside copper and silver. These elements share similar characteristics due to their placement in the same atomic group. Gold’s atomic structure is defined by its electron configuration, which is [Xe] 4f14 5d10 6s1. This configuration implies that gold has a full set of d-electrons in its penultimate shell and a single electron in its outermost shell.
This electron configuration contributes to gold’s excellent electrical conductivity, as the single 6s electron is relatively free to move, facilitating the flow of electric current. Furthermore the filled d-orbitals contribute to gold’s characteristic color and resistance to corrosion.
Gold Color and Optical Properties
Gold then has extremely interesting optical properties, because the nanoparticles change color from red to purple depending on their size. Other colors such as green and blue can be obtained by playing on the form factor, with more or less elongated nanoparticles.
Solid gold is a yellow metal, copper is red, and all other metals in their unoxidized state are gray. We can note that gold bars, or 24-carat jewelry being made of pure gold, appear very yellow.
The small variations in the color of gold come from the presence of another metal which forms an alloy: thus pink gold contains copper and white gold silver. More generally, the color of an object is essentially a phenomenon of light diffusion and depends on its ability to absorb light radiation at a given wavelength.
Solid gold absorbs blue wavelengths and therefore reflects the complementary color, yellow. This non-gray coloring of gold is explained by interband transitions whose energies correspond to photons of visible wavelength. Indeed, these photons can excite an electron from the 5d band to the 6s conduction band of the metal. The photons then tend to be absorbed by the metal.
Gold also exhibits excellent reflectivity, making it useful in applications such as heat shielding and reflective coatings for satellites and space suits.
Gold Chemical Reactivity
Pure gold is a noble metal (resistant to corrosion and discoloration), the most malleable and ductile of known metals, both dense and tender. It is a shiny yellow metal that does not oxidize in air or water. Gold remains untarnished when exposed to the atmosphere, retaining its luster. That’s why all the gold found in ancient treasures look as good as newly minted.
Its low reactivity is due to its high ionization energy and electron affinity, which make it hard for the metal to lose or gain electrons. Thus it does not easily form compounds with other elements under regular conditions.
Apart from its application in jewelry, gold finds industrial applications in odontology and electronics, due to its very good resistance to corrosion and its excellent electrical conductivity.
From a chemical point of view, gold is a transition metal capable of forming cations (mono and trivalents) in solution. It is less reactive than most other transition metals, but is attacked by aqua regia giving chloroauric acid HAuCl4, as well as by alkaline cyanide solutions, but not by hydrochloric acids HCl, nitric HNO3 nor sulfuric H2SO4.
Like lead, it dissolves in mercury forming a amalgam, but does not react with this metal. Gold being insoluble in nitric acid, which however dissolves silver and common metals, this property allows it to be separated and purify
Alloys and Compounds
If gold were used in its pure state in the manufacture of jewelry, the latter could be deformed at the slightest impact due to gold’s malleability. Therefore, jewelers combine gold with another precious metal with more resistant properties. The different alloys, whether natural or artificial, make it possible to resist corrosion, but also to change the color desired for the jewel.
A common formula is to use 18 carat gold, with jewelry composed of 75% pure gold and 25% alloy (silver, copper, platinum or palladium).
So-called artificial alloys include white gold, most often made with a palladium alloy. As for yellow gold, it is obtained by adding copper and silver (75% pure gold + 12.5% silver + 12.5% copper). Rose gold (75% pure gold + 5% silver + 20% copper) and red gold are obtained thanks to a copper content in the alloy. Purple gold is made up of gold (79%) and aluminum (21%).
Black gold is a color that is rarely found in jewelry. A base of white gold or an alloy of gold and cobalt followed by heat treatment must be used. This is also the case for blue gold. It is the heat treatment of the iron that gives this blue color to the jewelry.
Gold can form alloys with all other metals, which will alter its physical and chemical properties. Common alloying metals include silver, copper, and palladium. These alloys are used to enhance gold’s hardness and durability, making it more suitable for applications such as jewelry and coins (such as ancient coins).
Gold can also form compounds with various nonmetals, such as gold chloride (AuCl3) and gold oxide (Au2O3), which have applications in fields such as catalysis and nanotechnology.
Catalytic Properties
Although gold has long been neglected by chemists due to its inert nature, some experimental results published in 1973 showed that in highly divided form – the term nanometric was not in use 35 years ago – gold appeared to have catalytic properties.
But the scientific community’s interest in the chemical activity of gold arose after the demonstration by Masatake Haruta (Japan) in 1987, of the capacity of small gold nanoparticles to catalyze the oxidation reaction of carbon monoxide into carbon dioxide, even at room temperature.
Since then, gold nanoparticles has become the center of great scientific activity. They have demonstrated catalytic properties for numerous chemical reactions, such as the oxidation of alcohols to acids or aldehydes, the formation of hydrogen peroxide (peroxide), and the selective hydrogenation of C-C and N-O bonds.
Gold nanoparticles exhibit unique catalytic properties, and do not behave the same way as gold in normal form. These nanoparticles are able to catalyze numerous chemical reactions, such as oxidation and reduction processes, leading to active scientific research in the nanotechnology sector.
Gold’s catalytic properties also play a role in the production of chemicals and pharmaceuticals. For example gold catalysts are used in the synthesis of fine chemicals and the development of new drugs. Scientists can optimize these catalytic performance for specific chemical reactions, by modifying the size and shapes of the gold nanoparticules.
Gold Applications in Electronics
The main industrial use of gold is the electronics sector, where it is appreciated above all for its electrical conductivity. Gold’s combination of electrical conductivity, corrosion resistance and malleability makes it an ideal candidate for electronic components. Almost all electronic components contain a small amount of gold: smartphones, computers, televisions, etc.
The demand for gold from the telephone sector is measured in tens of tons as smartphone users renew their mobiles as new models get released. Paradoxically, the presence of these infinitesimal quantities of gold in electronic components leads to immense losses of gold for the economy. Hundreds of millions of phones are produced every year and each one of them contains around $0.50 worth of gold. The average lifespan of a mobile phone is around two years, and few of them are recycled at the end of their life.
Biomedical Applications
Gold’s biocompatibility and chemical properties, in particular gold nanoparticles, have sparked various new biomedical applications, including diagnostic tests, drug delivery systems, and medical imaging. Gold nanoparticles can be used in biology for imaging and diagnosis. They can also vectorize active substances such as drugs towards targets (organs, cells, etc.).
Thanks to their strong plasmonic absorbance, gold nanoparticles have great potential in the field of therapy. One of the techniques for recognizing cancer cells is the use of nanoparticles decorated with cancer cell recognition molecules such as antibodies or sugar-type ligands.
In the biological environment, these can recognize the antigens or receptors of cancer cells and thus send the nanoparticles more specifically to these cells. If the gold particles carry therapeutic compounds, then they can deposit them specifically into cancer cells.
Gold nanoparticles can also be used for diagnostic tests or rapid screening tests; for example, they are used in some pregnancy tests.
Concentrated colloidal suspensions of gold nanoparticles are very colorful even at low concentrations. It is therefore possible to use them for visual tests using their color. The principle of the pregnancy test is to detect in the urine the presence of a hormone produced only during pregnancy, chorionic gonadotrophic hormone, noted beta-HCG.
For this, it is possible to use gold nanoparticles functionalized on the surface with anti-beta-HCG antibodies. When the pregnancy hormone is present in the urine, the gold nanoparticles will then bind to it via the antibody.
When carrying out the pregnancy test, the urine carries gold particles which are retained on the test strip only if beta-HCG is present. This creates a colored red band which corresponds to the accumulation of particles. In reality, in pregnancy tests, there are always two bands observed: one band linked to the presence of the hormone (the test band), the second band simply being a control band allowing you to verify that the test has been correctly carried out.
Gold nanoparticles can also help identify cancer cells using photoacoustic imaging.
Photoacoustic imaging, as a modality of biomedical imaging, is based on the photoacoustic effect. In photoacoustic imaging, non-ionizing laser pulses are sent into biological tissues into which gold particles are previously injected. These particles have the capacity to absorb part of this light and create a strong release of heat.
Consequently, part of the energy emitted by the laser is then converted into heat. This local increase in heat creates an expansion of the tissues. The latter will undergo expansion-compression cycles generating mechanical waves which can then be detected and analyzed to construct an internal image of the patient.
Since biological tissues do not have the same optical absorption, it is possible to produce 2D or 3D images of the targeted area. Gold particles, and more particularly gold nanorods, have very strong plasmonic absorbance, which is why they are excellent candidates as contrast agents in photoacoustic imaging.